
Summarized by Howard Velasquez, BS.CE., M.ASCE,
These ISO standards, TR and TS (abbreviated titles) are only a core collection of several hundreds of standards available for the oil & gas industry from ABNT, NSI, API, AS, BSI, CSA, NORSOK, NF, GOST, SAC etc. Some ISO/TC67 standards have been withdrawn and the relevant API standard is referenced above.
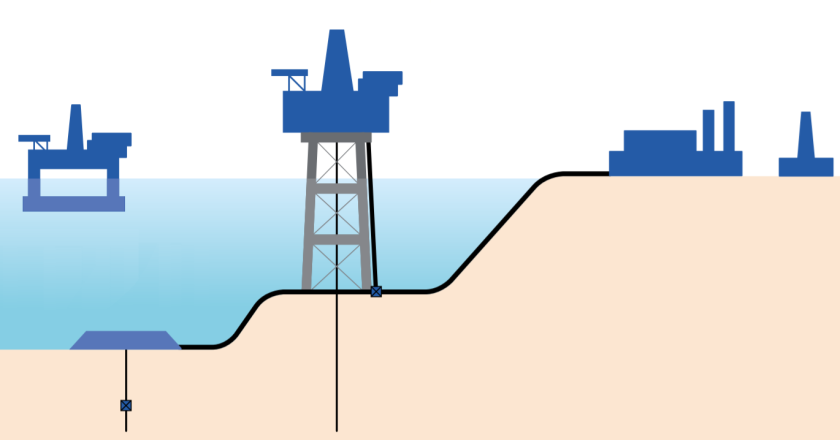
ISO 10418 Process safety systems (Rev)
ISO 10419 Replaced by API Spec 6AV2
ISO 10423 Wellhead & Christmas tree equipment
ISO 12489 Reliability modeling/safety systems
ISO 13354 Shallow gas diverter equipment
ISO 13533 Drill-through equipment (BOPs)
ISO 13534 Hoisting equipment – care/maintenance
ISO 13535 Hoisting equipment – specification
ISO 13626 Drilling and well-servicing structures
ISO 13702 Control and mitigation of fires and explosions
ISO 13703 Offshore piping systems
ISO 14224 Reliability and maintenance data (Rev)
ISO 14692 GRP piping, Parts 1-4 (Rev)
ISO 14693 Drilling equipment
ISO 15138 Heating, ventilation, and air-conditioning (Rev)
ISO 15156 Cracking-resistant materials for use in H2S environments, Parts 1-3
ISO 15544 Emergency response
ISO 15663 Life cycle costing, Parts 1–3
ISO 16901 Risk assessment in the design of onshore LNG installations
ISO 16903 Characteristics of LNG influencing design and material selection
ISO 16904 LNG Marine Transfer Arms (New)
ISO 17177 Unconventional LNG transfer systems
ISO 17292 Metal ball valves
ISO 17776 Major Accident hazard management during design (Rev)
ISO 17781 Duplex stainless steel materials testing requirements (New)
ISO 17782 Qualification of manufacturers of special materials (New)
ISO 17945 Materials resistant to sulfide stress cracking
ISO 17969 Guidelines on competence for personnel (Rev)
ISO 18683 Systems and installations for supply of LNG as fuel to ships
ISO 19008 Standard Cost Coding System (New)
ISO 20815 Production assurance and reliability management
ISO 21457 Materials selection
ISO 23936-1 Thermoplastics
ISO 23936-2 Elastomers
ISO 27469 Method of test for offshore fire dampers
ISO 29001 Sector-specific quality management systems
ISO 13624 Marine drilling riser systems, Parts 1-2
ISO 13625 Marine drilling riser couplings
ISO 19901-7 Station keeping systems
ISO 10855 Offshore containers, Part 1-3 (New)
ISO 18647 Modular drilling rigs for offshore fixed platforms (New)
ISO 18797-1 Elastomeric coating of risers – polychloroprene or EPDM (New)
ISO 19900 General requirements for offshore structures
ISO 19901-1 Metocean design and operating considerations
ISO 19901-2 Seismic design procedures and criteria (Rev)
ISO 19901-3 Topsides structure
ISO 19901-4 Geotechnical and foundation design (Rev)
ISO 19901-5 Weight control (Rev)
ISO 19901-6 Marine operations
ISO 19901-8 Marine soil investigations
ISO 19902 Fixed steel offshore structures
ISO 19903 Fixed concrete offshore structures (Rev)
ISO 19904-1 Monohulls, semi-submersibles and spars (Rev)
ISO 19905-1 Site-specific assessment of jack-ups (Rev)
ISO 19905-2 Jack-ups commentary
ISO 19905-3 Site-specific assessment of floating units (New)
ISO 19906 Arctic offshore structures
ISO 35101 Arctic Operations – Working environment (New)
ISO 35103 Arctic Operations – Environmental monitoring (New)
ISO 35104 Arctic operations – Ice management (New)
ISO 35106 Arctic Metocean, ice and seabed data (New)
ISO 3977-5 Gas turbines – procurement
ISO 10428 Sucker rods
ISO 10431 Pumping units
ISO 10434 Bolted bonnet steel gate valves
ISO 10436 Replaced by API Std 611
ISO 10437 Special-purpose steam turbines
ISO 10438 Lubrication, shaft-sealing and control-oil systems, Parts 1–4
ISO 10439 Centrifugal compressors
ISO 10440-1 Rotary-type positive-displacement process compressors (oil-free)
ISO 10440-2 Rotary PD packaged air compressors
ISO 10441 Flexible couplings – special
ISO 10442 Integrally geared air compressors
ISO 12211 Spiral plate heat exchangers
ISO 12212 Hairpin heat exchangers
ISO 13631 Reciprocating gas compressors
ISO 13691 High speed enclosed gear units
ISO 13704 Calculation of heater tube thickness
ISO 13705 Fired heaters for general service
ISO 13706 Air-cooled heat exchangers
ISO 13707 Reciprocating compressors
ISO 13709 Centrifugal pumps
ISO 13710 Reciprocating positive displacement pumps
ISO 14691 Flexible couplings – general
ISO 15547 Heat exchangers, Parts 1-2
ISO 15649 Piping
ISO 15761 Steel valves DN 100 and smaller
ISO 16812 Shell & tube heat exchangers
ISO 16901 Risk assessment of onshore LNG installations
ISO 16961 Internal coating and lining of steel storage tanks
ISO 17177 Unconventional LNG transfer systems
ISO 17292 Metal ball valves
ISO 17348 Materials Selection in CO2 Environment for casing, tubing and downhole equipment (New)
ISO 17349 Streams containing high levels of CO2 (New)
ISO 18796-1 Internal coating and lining of process vessels (New)
ISO 18624-1 Design and testing of LNG storage tanks
ISO 20088-1 Resistance to cryogenic spillage of insulation materials – Liquid phase (New)
ISO 21049 Centrifugal and rotary pumps shaft sealing
ISO 23251 Replaced by API Std 521
ISO 24817 Composite repairs for pipework (Rev)
ISO 25457 Flares details
ISO 27509 Compact flanged connections
ISO 28300 Venting of storage tanks
ISO 28460 LNG – Ship to shore interface
ISO 13628-1 Subsea production systems
ISO 13628-2 Subsea flexible pipe systems
ISO 13628-3 Subsea TFL pumpdown systems
ISO 13628-4 Subsea wellhead and tree equipment
ISO 13628-5 Subsea control umbilical
ISO 13628-6 Subsea production controls
ISO 13628-7 Completion/workover riser system
ISO 13628-8 ROT and interfaces
ISO 13628-9 ROT intervention systems
ISO 13628-10 Bonded flexible pipe
ISO 13628-11 Flexible pipe systems for subsea and marine applications
ISO 13628-15 Subsea structures and manifolds
ISO 10400 Calculations for OCTG performance properties
ISO 10405 Care/use of casing/tubing
ISO 10407-1 Drill stem design
ISO 10407-2 Inspection and classification of drill stem elements
ISO 10414-1 Field testing of water-based fluids
ISO 10414-2 Field testing of oil-based drilling fluids
ISO 10416 Drilling fluids – lab testing
ISO 10417 Subsurface safety valve systems
ISO 10422 Replaced by API Spec 5B
ISO 10424-1 Rotary drill stem elements
ISO 10424-2 Threading and gauging of connections
ISO 10426-1 Well cementing
ISO 10426-2 Testing of well cements
ISO 10426-3 Testing of deepwater well cement
ISO 10426-4 Atmospheric foamed cement slurries
ISO 10426-5 Shrinkage and expansion of well cement
ISO 10426-6 Static gel strength of cement formulations
ISO 10427-1 Bow spring casing centralizers
ISO 10427-2 Centralizer placement and stop-collar testing
ISO 10427-3 Performance testing of cement float equipment
ISO 10432 Subsurface safety valves
ISO 10433 Replaced by API Spec 6AV1
ISO 11960 Casing and tubing for wells
ISO 11961 Drill pipe
ISO 12835 Qualification of casing connections for thermal wells
ISO 13085 Tubing aluminum alloy pipes
ISO 13500 Drilling fluids
ISO 13501 Drilling fluids – processing systems evaluation
ISO 13503-1 Measurement of viscous properties of completion fluids
ISO 13503-2 Measurement of properties of proppants
ISO 13503-3 Testing of heavy brines
ISO 13503-4 Measurement of stimulation & gravel-pack fluid leak-off
ISO 13503-5 Measurement of long term conductivity of proppants
ISO 13503-6 Measuring leak-off of completion fluids under dynamic conditions
ISO 13678 Thread compounds
ISO 13679 Casing and tubing connections testing
ISO 13680 CRA seamless tubes for casing & tubing
ISO 14310 Packers and bridge plugs
ISO 14998 Accessory completion equipment
ISO 15136 Progressing cavity pump systems, Parts 1-2
ISO 15463 Field inspection of new casing, tubing, and plain end drill pipe
ISO 15464 Gauging and inspection of threads
ISO 15551-1 Electric submersible pump systems for artificial lift
ISO 15546 Aluminium alloy drill pipe
ISO 16070 Lock mandrels and landing nipples
ISO 16530-1 Well Integrity life cycle governance manual (New)
ISO 16530-2 Well integrity operational phase
ISO 17078-1 Side-pocket mandrels
ISO 17078-2 Flow control devices for side-pocket mandrels
ISO 17078-3 Latches & seals for side-pocket mandrels & flow control devices
ISO 17078-4 Side-pocket mandrels and related equipment
ISO 17824 Sand control screens
ISO 20312 Design of aluminium drill string
ISO 27627 Aluminium alloy drill pipe thread gauging
ISO 28781 Subsurface tubing mounted formation barriers
ISO 3183 Steel pipe for pipeline transportation systems
ISO 12490 Actuation, mechanical integrity and sizing for pipeline valves
ISO 12736 Wet thermal insulation coatings
ISO 12747 Pipeline life extension
ISO 13623 Pipeline transportation systems (Rev)
ISO 13847 Welding of pipelines
ISO 14313 Pipeline valves
ISO 14723 Subsea pipeline valves
ISO 15589-1 Cathodic protection of on-land pipelines
ISO 15589-2 Cathodic protection for offshore pipelines
ISO 15590-1 Pipeline induction bends
ISO 15590-2 Pipeline fittings
ISO 15590-3 Pipeline flanges
ISO 16440 Steel cased pipelines (New)
ISO 16708 Pipeline reliability-based limit state design
ISO 19345-1 Life cycle integrity management for onshore pipeline
ISO 21329 Test procedures for pipeline mechanical connectors
ISO 21809-1 Polyolefin coatings (3-layer PE and 3-layer PP)
ISO 21809-2 Fusion-bonded epoxy coatings
ISO 21809-3 Field joint coatings (Rev)
ISO 21809-4 Polyethylene coatings (2-layer PE)
ISO 21809-5 External concrete coatings (Rev)